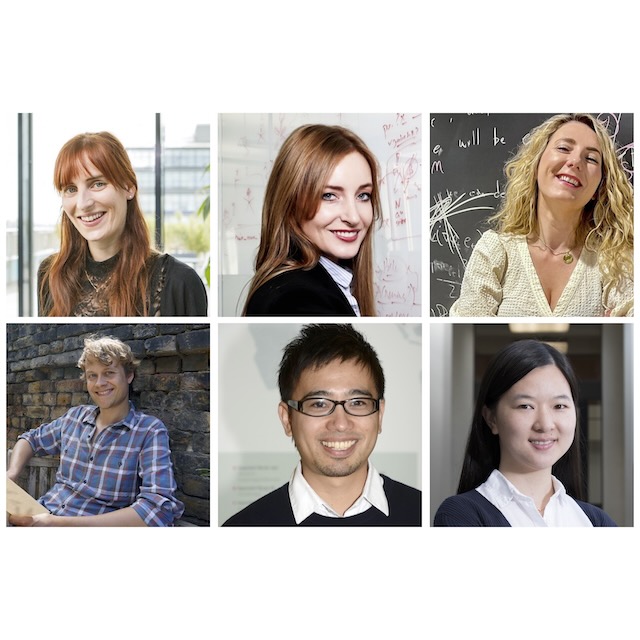
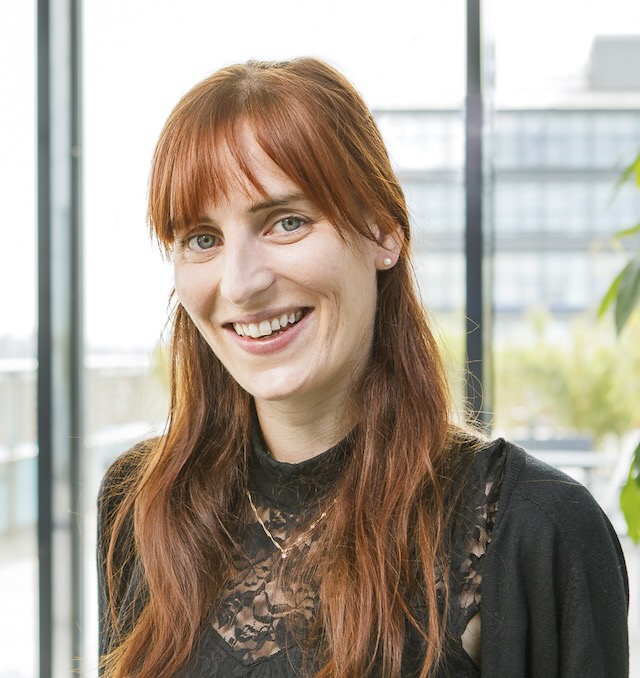
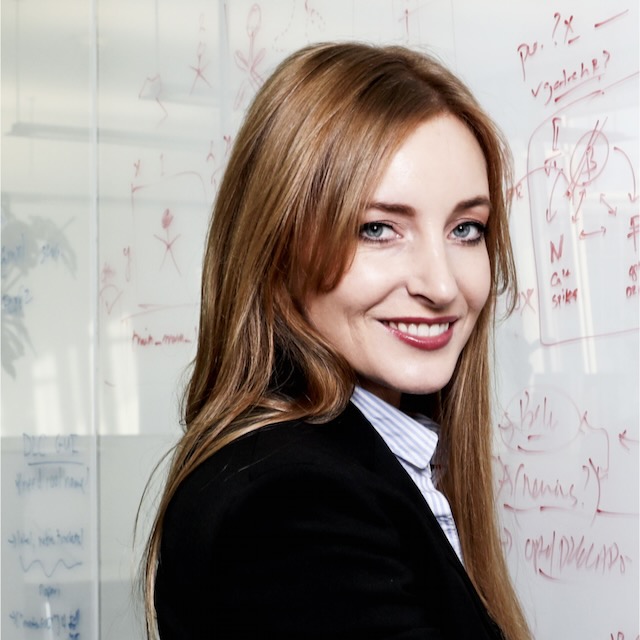
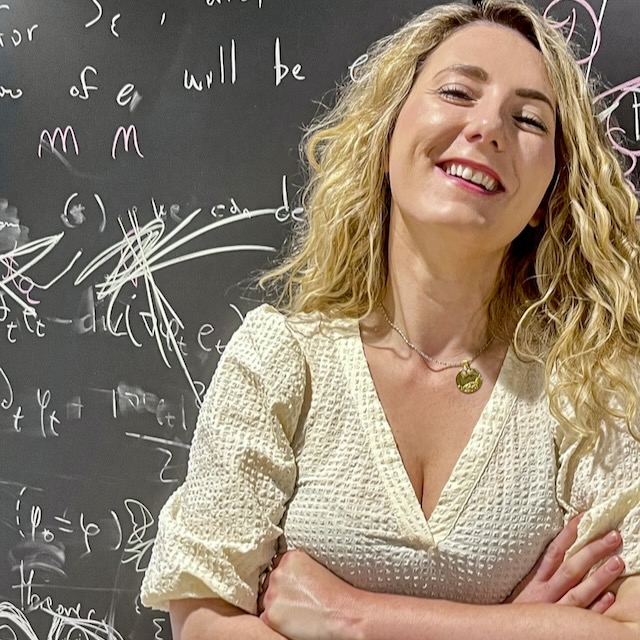
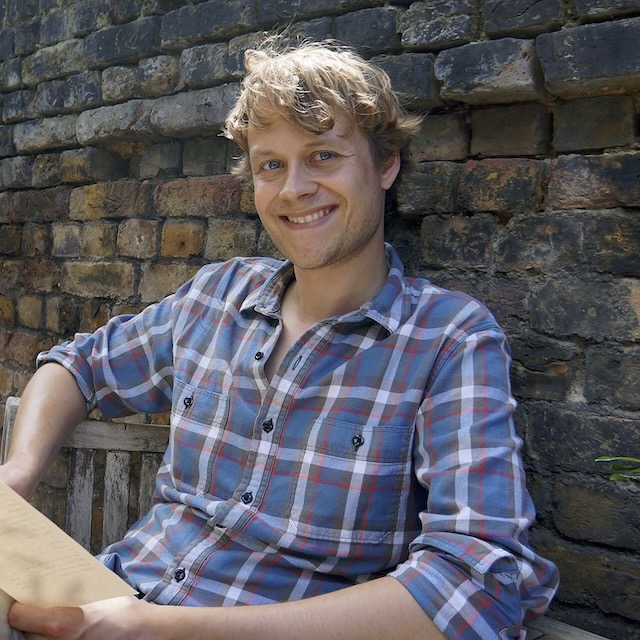
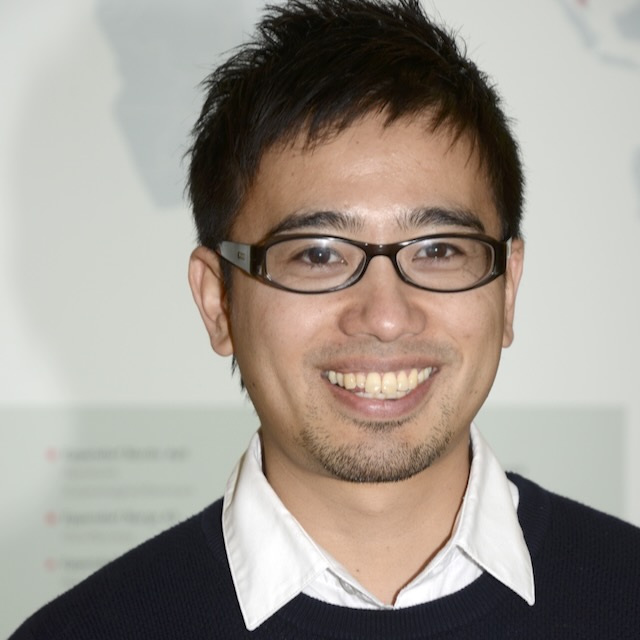
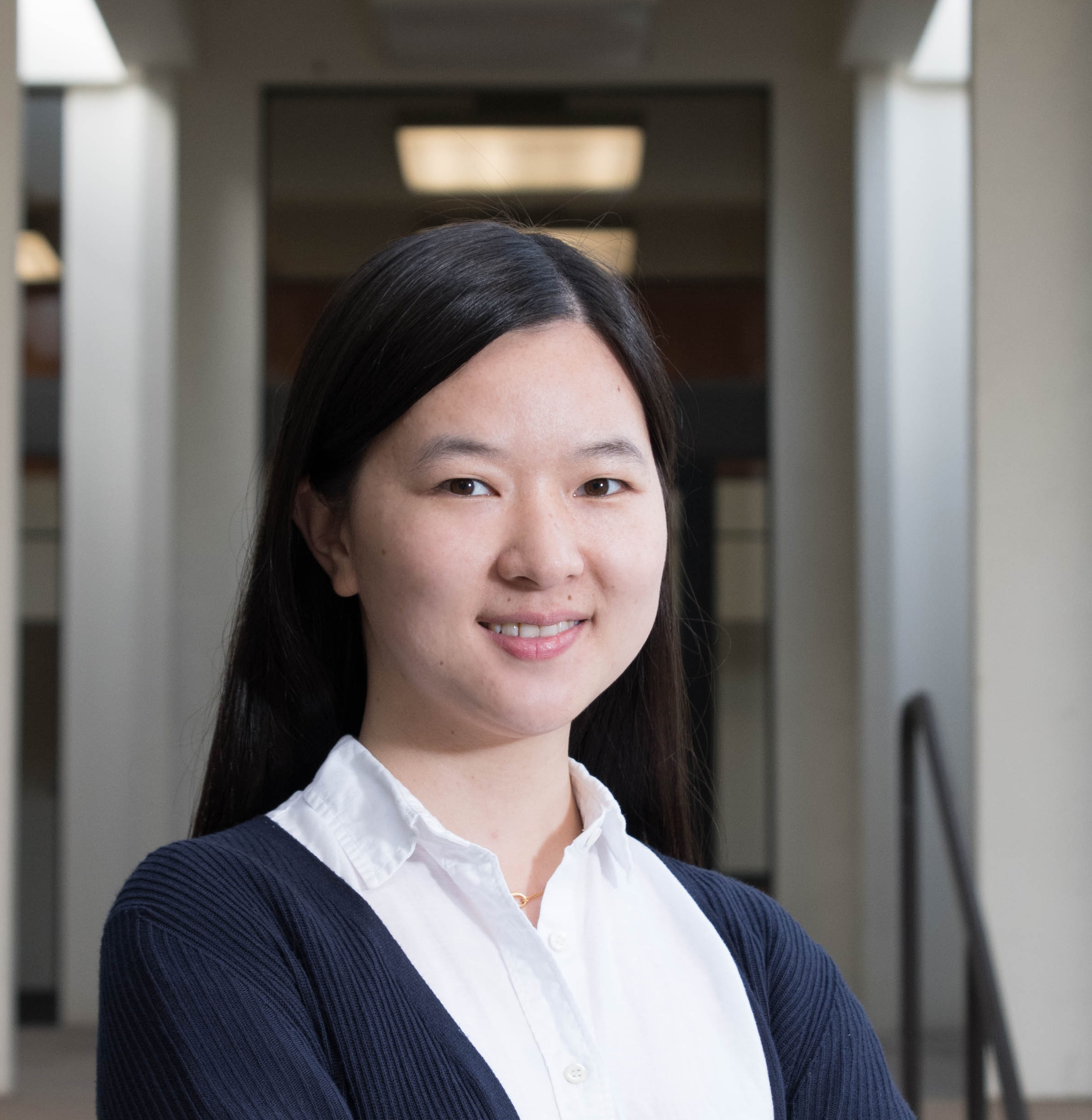
2022 Vallee Scholars
The Vallee Foundation is delighted to announce the six 2022 Vallee Scholars. The Vallee Scholar program continues to provide unrestricted funding for national and international early career researchers at a critical stage in their tenure-track careers. As in the past, the quality of the candidates nominated this year was exceptional making the final selections difficult. In addition to the quality of their science and potential impact of their proposed research, the Committee considered each applicant's career trajectory and their commitment to fostering a diverse work environment. The 2022 Vallee Scholars and the description they have provided of their science interests are below.
Stephanie Ellis, PHD, Assistant Professor, Max Perutz Labs, University of Vienna.
Mammalian female cells have two X chromosomes; male cells have only a single X chromosome. To be functionally equal to male cells, each female cell expresses genes from just one of two X-chromosome; the other X-chromosome is permanently deactivated early in development. As a result, female tissues are a patchwork of cells with two different genotypes – about half the cells express genes from the maternally-inherited X-chromosome, whereas the other half express genes from the paternally-inherited X-chromosome. Occasionally, mutations carried on one X-chromosome skew tissue composition such that there are more cells that have a particular X-chromosome active than the other. When X-linked mutations also cause disease, the extent of X-skewing is clinically important and significantly impacts patient outcomes. X-skewing hints at the tantalizing possibility of a cellular survival-of-the-fittest selection process. The central goal of my project is to molecularly unmask this selection process. My studies use sophisticated genetics and microscopy techniques to map patterns of X-chromosome activation in healthy and diseased tissues, and to identify molecules that control these distribution patterns. Ultimately, I will leverage my findings to develop therapies for myriad devastating X-linked genetic diseases that predominantly affect women, and for which there are no current treatments.
Mackenzie Mathis, PhD, the Bertarelli Foundation Chair of Integrative Neuroscience and an Assistant Professor at the Swiss Federal Institute of Technology, Lausanne (EPFL).
Research animals are the workhorses of modern biology, medicine, and neuroscience. For example, nearly 25 million rodents are used in the USA annually. Yet, our actual understanding of the ethological "umwelt" of the laboratory mouse is lacking. While countless studies use them and analyze their behavior in short bouts (i.e., task settings such as open field assays, rotarod, or decision-making behaviors), a true understanding of the baseline "life of a lab mouse" remains unreported---arguably as analyzing behavior is hard and time consuming. Yet, understanding their ecological behavior is of great importance for the study of health and disease in preclinical medicine. In this proposal, I aim to develop novel algorithms in order to aid in our quest to understanding natural behavior. While the deep-learning tools I aim to develop are universal (no constraints on the type of animal used), here we will develop and deploy them on a large-scale dataset of mice in extended 3D home-cages.
Anđela Šarić, PhD, Assistant Professor at the Institute of Science and Technology Austria.
Cell division, the process in which one cell splits into two, is essential for the existence of life. To divide its material, the cell organizes its proteins into long rope-like assemblies that wrap around the cell waist from the inside. These assemblies then pinch the cell waist until it is split into two. One particular family of proteins, called ESCRT, drive this process in a range of different cells. The same proteins also repair cell surface when it is wounded by chemical or mechanical injury. How protein assemblies produce mechanical work to pinch the cell into two, and how they can also repair cells, is not understood. These processes are notoriously complicated in living cells. Luckily, they must obey the laws of physics and chemistry. Here I will develop computer models, rooted in physics and chemistry, to capture cell division and cell repair by ESCRT assemblies in different forms of life. By comparing my results with laboratory experiments, I will determine how cell division and repair take place and which characteristics of the system control it. The in-depth understanding of cell reshaping principles that my research will provide can allow us to design therapeutics for the cases when these processes go wrong and lead to diseases. It can also instruct us on how to reproduce such processes in laboratory to make artificial tissues and smart bio-materials.
Peter H Sudmant, PhD, Assistant Professor of Integrative Biology at the University of California Berkeley. Age is the primary risk factor for almost all common human diseases including cancers, cardiovascular disease, diabetes, and neurodegenerative conditions. Simultaneously, lifespan varies by more than three orders of magnitude among vertebrates, however, the underlying genetic determinants of this vast range in lifespan variation remain unclear. Our lab seeks to identify novel pathways and targets to mitigate diseases of human aging by probing the critical adaptations that have shaped lifespan across the diversity of vertebrate species. Here we propose to examine the extreme variation in lifespan observed in bats. Bats are exceptionally long lived given their size and exhibit remarkably low incidence of cancer, a unique feature among organisms. The adaptations that facilitate these characteristics are also relevant to human health given that bats are mammals. We propose to generate complete genomes of closely related species of bat using state-of-the-art methods. Computational approaches will be used to identify genes associated with extreme lifespan. We will generate cellular models from these species and use CRISPR to characterize candidate genes and perform a large-scale screen to identify pathways associated with stress-tolerance and cancer resilience in bats. Together this research will provide unique insights into the molecular underpinnings of aging and disease.
Shigeki Watanabe, PhD, Associate Professor in the Department of Cell Biology at the Johns Hopkins University. Biological forms are complex yet beautiful, with diverse forms of cells underlying specialized functions. For information processing, nerve cells, or neurons, in the brain possess ultrathin cables that contact many target cells to send and receive signals. These thin cables are essential for conducting electrical signals over a long range. However, their shape constantly changes to allow for complex and diverse computations. Moreover, these cables are highly unstable. Imagine stretching a piece of chewing gum, it will spontaneously break as it becomes too thin. The cables of our neurons are similarly vulnerable and must be made resistant to the breakage. We recently discovered that the physical environment (i.e. salt and sugar concentrations in cerebrospinal fluid) and materials used to make these ultrathin cables (i.e. lipids, skeletal proteins) are essential to their stability. In devastating neurological disorders, many of these components are dysregulated, leading to the loss of cables and eventual neuronal death (neurodegeneration). Thus, it is essential to understand how these factors are normally controlled based on neuronal activity to accommodate diverse forms of cables and how they are dysregulated in disease. In this proposal, we aim to reveal the interrelationship among force, form and neuronal function.
Lu Wei, PhD, Assistant Professor of Chemistry in the Division of Chemistry and Chemical Engineering, at the California Institute of Technology. The ability to systematically investigate the complex brain metabolism at subcellular level offers rich information on how the chemical activities cooperate with normal brain functions and how they become deregulated under diseased conditions. Here, we aim to integrate two chemical imaging techniques that the PI pioneered with the adoption of existing powerful tools (e.g. optogenetics) into a functional optical imaging platform for interrogation of brain metabolism across space, time and cell types. First, we aim to understand the dynamical metabolism in live neurons and brain tissues to generate metabolic hot-maps. Second, we will correlate the identified spatial metabolic hot-maps with cell type markers. Third, with the optogenetic tools, we will repetitively fire the action potential of one neuron and track the metabolic integration within this neuron and in surrounding cells to understand the integration of temporal information from electrical signal to metabolic changes and to the influence on circuit rewiring. Ultimately, we will move the study to disease mouse models to offer potential new insights on the metabolic dysregulation. Our proposed study should generate valuable systems-level information of metabolism in the mammalian brain at subcellular level. Since most of the metabolites come from our food, ultimately, it may tell us what we eat may influence how we think.